Batteries for electric cars Part 2: The potential of the anode
Today, the cathode material is the focus of the discussion on cell chemistry. What is overlooked is how great the potential is at the anode.
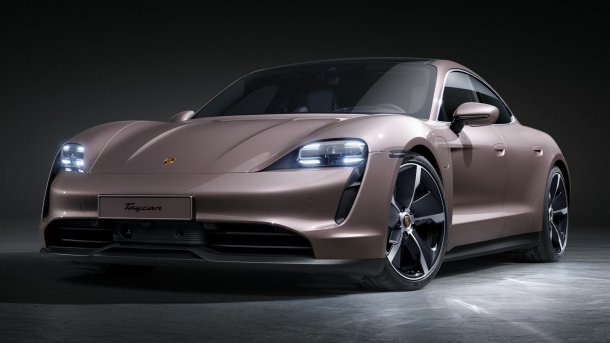
The Porsche Taycan does not have a pure graphite anode, but a silicon admixture in the single-digit percentage range. Almost all other electric cars have only graphite on the anode. If it were possible to add more and more silicon, the energy density and charging speed would improve significantly.
(Bild: Porsche)
- Christoph M. Schwarzer
(Hier finden Sie die deutsche Version des Beitrags)
For many decades, the focus in the further development of the powertrain in cars was on the engine. With the switch to battery electric drive, this is shifting, as the battery cell is now the focus of interest. Huge sums are currently being invested globally to achieve progress in this area. Energy density, durability, charging performance and, above all, costs must be optimized if the battery-electric drive is to stand a chance anywhere in the world.
In a series of articles, we want to show where we are today and where the development is heading. The European Union has set the political framework: From January 1, 2035, only passenger cars with no direct CO₂ emissions may be newly registered. According to current estimates, this will largely be battery-electric cars. Because the industry cannot simply be switched on and off, there will be a continuous ramp-up in the almost twelve years until the deadline.
The discussion about the cell chemistry of traction batteries mostly revolves around the cathode material. The two main trends: Firstly, the mixing ratio for cathodes with nickel, manganese and cobalt (NMC) is changing - more and more nickel and less and less cobalt is being used. On the other hand, the market share of lithium iron phosphate (LFP) cells, the only relevant alternative to NCM, is currently rising steadily. Perhaps further optimization can be achieved through modifications. However, the potential of the anode is largely overlooked in the public debate: It is here, and not at the cathode, that the possibility of achieving a new quality lies. What is at stake with anodes made of graphite, silicon, lithium and, as a transition to the next part of our battery series, hard carbon?
Graphite
Almost all electric cars have battery cells with a graphite anode. The mineral has proven itself. Graphite is reliable, why change what works wonderfully? The disadvantages: Graphite is a limiting factor in charging speed. It is heavy and consequently a culprit in low energy density. There is great dependence on China for extraction, where over 90 percent of graphite is mined.
"The discovery that graphite is suitable as an anode material earned the Japanese Akira Yoshino the Nobel Prize in 2019," explains Prof. Dr. Markus Hölzle from the Center for Solar Energy and Hydrogen Research Baden-Württemberg (ZSW). Graphite is so interesting, he said, because it is actually unstable, but forms a protective layer on the surface during operation, in other words, it is passivated. "Today, graphite has been researched out, but it remains unsurpassed in series application," Hölzle notes.
Silicon
From a down-to-earth perspective, the greatest potential for improving the anode and thus the cell itself lies in silicon. It is well known that silicon works as an anode material. Mathematically, it can absorb ten times as much lithium as graphite. For every atom of silicon, four lithium atoms can be absorbed during charging and released again during discharging. As a result, the volume expands considerably and shrinks again. "This breathing leads to the detachment of silicon particles in the medium term," says Prof. Hölzle of ZSW. "This is counteracted by adding silicon to only part of the graphite." This admixture can already be found in the low single-digit percentage range, for example in the Porsche Taycan. Mercedes says on request that the electric G-Class could be equipped with it from the middle of the decade.
(Bild: Volkswagen)
A mixed electrode is better than exclusively with graphite and worse than with pure silicon. On the other hand, such an electrode hardly "breathes" in volume. It allows a doubling in specific lithium absorption and half the thickness of the battery. "Thin electrodes are generally easier and faster to charge because the lithium doesn't have to travel long distances first," explains Markus Hölzle of ZSW. Because silicon is much lighter than graphite, the energy density automatically increases significantly.
Industry circles assume that an admixture of up to 20 percent silicon is feasible for series production. Should this actually succeed, an anode made of silicon and graphite will be the toughest competitor for the solid-state cell, because the energy density goes in a similar direction, but the manageability is much easier and the costs in production are consequently lower.
Lithium
Solid-state cells, often referred to as "solid-state batteries," are considered to be the final goal of development. Volkswagen's chief battery developer, Frank Blome, spoke of the "end game. What is meant is the electrolyte, which is no longer liquid but solid. However, the definition is not clear and currently includes everything between gel, soft powders and rigid ceramics, i.e. also semi-solid electrolytes.
Only a few companies are researching actual solid-state cells, such as Quantumscape, where again Volkswagen is involved. Only here is the core of the solid-state battery at stake: A solid electrolyte is the prerequisite for installing an anode made of pure lithium. Solid state is therefore not an end in itself, but the material for implementing the metallic lithium anode.
(Bild: Volkswagen)
Metallic lithium is highly reactive. A pure lithium anode is a very thin sheet. If it is possible to produce a metallic lithium anode in series, this will result in the highest energy density ever. The solid electrolyte is intended to prevent the biggest problem of this setup, namely dendrite growth - the spiky growth of lithium - which leads to short-circuiting and fire. Consequently, only non-flammable materials such as solid electrolytes may be used.
Professor Hölzle of ZSW classifies the pure lithium anode: "These solid-state cells are difficult to build and operate because the lithium must now move through a practically solid electrolyte. Good electrolytes are complex and expensive." It is therefore considered likely that solid-state cells will first be sold in very expensive luxury sedans or sports cars. If anodes with silicon admixtures could offer almost the same properties for much less money, that would be the end of the real solid-state battery with a metallic lithium anode.
(Bild: CATL)
Hard Carbon
Hard carbon leads into the next part of our battery series. It is the anode material that will be used when lithium is replaced by sodium. The world market leader in battery cells, CATL, is already promising series production of the amorphous carbon for this year. This much in advance: sodium is cheaper than lithium. If mass motorization is to work internationally with battery electric vehicles, cost is extremely important. The decisive key will be to get these under control.
(mfz)